IRF3 prevents colorectal tumorigenesis via inhibiting the nuclear translocation of β-catenin
- PMID: 33188184
- PMCID: PMC7666182
- DOI: 10.1038/s41467-020-19627-7
IRF3 prevents colorectal tumorigenesis via inhibiting the nuclear translocation of β-catenin
Erratum in
-
Author Correction: IRF3 prevents colorectal tumorigenesis via inhibiting the nuclear translocation of β-catenin.Nat Commun. 2023 Nov 29;14(1):7862. doi: 10.1038/s41467-023-43761-7. Nat Commun. 2023. PMID: 38030635 Free PMC article. No abstract available.
Abstract
Occurrence of Colorectal cancer (CRC) is relevant with gut microbiota. However, role of IRF3, a key signaling mediator in innate immune sensing, has been barely investigated in CRC. Here, we unexpectedly found that the IRF3 deficient mice are hyper-susceptible to the development of intestinal tumor in AOM/DSS and Apcmin/+ models. Genetic ablation of IRF3 profoundly promotes the proliferation of intestinal epithelial cells via aberrantly activating Wnt signaling. Mechanically, IRF3 in resting state robustly associates with the active β-catenin in the cytoplasm, thus preventing its nuclear translocation and cell proliferation, which can be relieved upon microbe-induced activation of IRF3. In accordance, the survival of CRC is clinically correlated with the expression level of IRF3. Therefore, our study identifies IRF3 as a negative regulator of the Wnt/β-catenin pathway and a potential prognosis marker for Wnt-related tumorigenesis, and describes an intriguing link between gut microbiota and CRC via the IRF3-β-catenin axis.
Conflict of interest statement
The authors declare no competing interests.
Figures
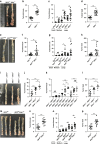
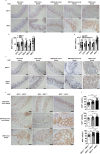
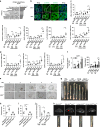
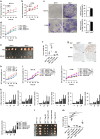
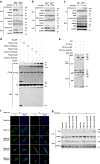
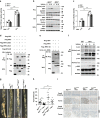
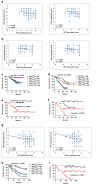
Similar articles
-
TRIB3 Interacts With β-Catenin and TCF4 to Increase Stem Cell Features of Colorectal Cancer Stem Cells and Tumorigenesis.Gastroenterology. 2019 Feb;156(3):708-721.e15. doi: 10.1053/j.gastro.2018.10.031. Epub 2018 Oct 24. Gastroenterology. 2019. PMID: 30365932
-
RNF6 promotes colorectal cancer invasion and migration via the Wnt/β-catenin pathway by inhibiting GSK3β activity.Pathol Res Pract. 2021 Sep;225:153545. doi: 10.1016/j.prp.2021.153545. Epub 2021 Jul 10. Pathol Res Pract. 2021. PMID: 34352441
-
TTPAL Promotes Colorectal Tumorigenesis by Stabilizing TRIP6 to Activate Wnt/β-Catenin Signaling.Cancer Res. 2019 Jul 1;79(13):3332-3346. doi: 10.1158/0008-5472.CAN-18-2986. Epub 2019 Apr 24. Cancer Res. 2019. PMID: 31018940
-
Interaction of the Wnt/β-catenin and RAS-ERK pathways involving co-stabilization of both β-catenin and RAS plays important roles in the colorectal tumorigenesis.Adv Biol Regul. 2018 May;68:46-54. doi: 10.1016/j.jbior.2018.01.001. Epub 2018 Jan 10. Adv Biol Regul. 2018. PMID: 29449169 Review.
-
Therapeutic potential of targeting the Wnt/β-catenin signaling pathway in colorectal cancer.Biomed Pharmacother. 2019 Feb;110:473-481. doi: 10.1016/j.biopha.2018.11.082. Epub 2018 Dec 6. Biomed Pharmacother. 2019. PMID: 30530050 Review.
Cited by
-
Comprehensive Analysis of MICALL2 Reveals Its Potential Roles in EGFR Stabilization and Ovarian Cancer Cell Invasion.Int J Mol Sci. 2023 Dec 30;25(1):518. doi: 10.3390/ijms25010518. Int J Mol Sci. 2023. PMID: 38203692 Free PMC article.
-
Toll-Like Receptors Serve as Biomarkers for Early Diagnosis and Prognosis Assessment of Kidney Renal Clear Cell Carcinoma by Influencing the Immune Microenvironment: Comprehensive Bioinformatics Analysis Combined With Experimental Validation.Front Mol Biosci. 2022 Jan 21;9:832238. doi: 10.3389/fmolb.2022.832238. eCollection 2022. Front Mol Biosci. 2022. PMID: 35127830 Free PMC article.
-
IRF3 Knockout Results in Partial or Complete Rejection of Murine Mesothelioma.J Clin Med. 2021 Nov 7;10(21):5196. doi: 10.3390/jcm10215196. J Clin Med. 2021. PMID: 34768716 Free PMC article.
-
The multiple roles of interferon regulatory factor family in health and disease.Signal Transduct Target Ther. 2024 Oct 9;9(1):282. doi: 10.1038/s41392-024-01980-4. Signal Transduct Target Ther. 2024. PMID: 39384770 Free PMC article. Review.
-
Blood-based DNA methylation in advanced Nasopharyngeal Carcinoma exhibited distinct CpG methylation signature.Sci Rep. 2023 Dec 12;13(1):22086. doi: 10.1038/s41598-023-45001-w. Sci Rep. 2023. PMID: 38086861 Free PMC article.
References
Publication types
MeSH terms
Substances
LinkOut - more resources
Full Text Sources
Medical
Molecular Biology Databases